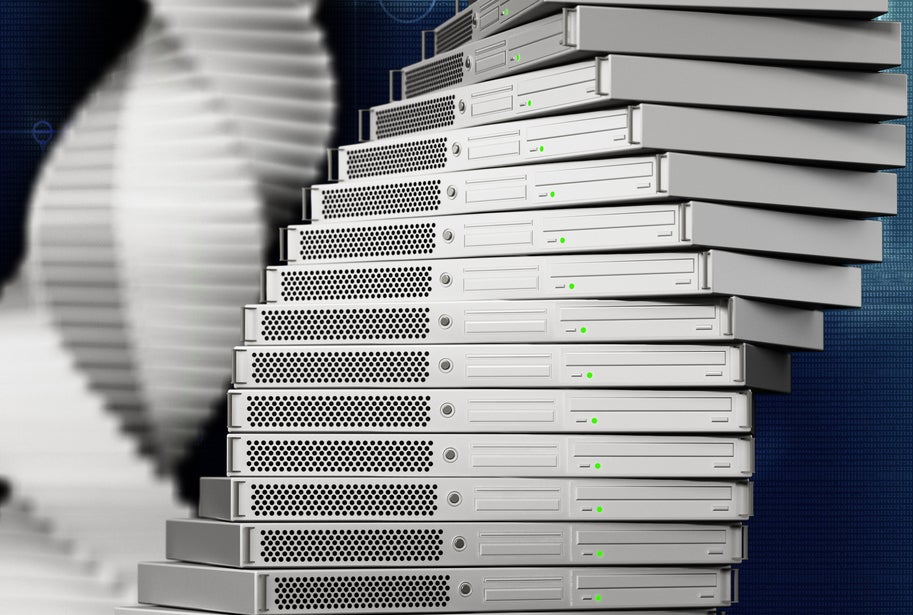
German Netflix science thriller Biohackers follows medical student Mia Akerlund at the University of Freiburg, who is not only pursuing a degree, but also investigating the dark secrets of the university’s star lecturer Professor Tanja Lorenz. The show explores the themes of synthetic biology and genetic engineering – and the consequences if these tools get into the wrong hands – as Akerlund investigates the role Lorenz played in her brother’s and parents’ deaths many years prior.
To demonstrate the possibilities of so-called biohacking and to illustrate that some of the show’s concepts are more than just science fiction, Netflix has partnered with ETH Zurich’s Professor Dr Robert Grass to store the first episode of Biohackers in synthetic DNA. Timed with the launch of Biohackers at the end of August, this makes the show the first Netflix Original Series to be stored in DNA.
Grass, in turn, reached out to a previous partner of his, San Francisco-based synthetic biology company Twist Bioscience, to supply the physical synthetic DNA segments. This collaboration leverages Twist’s high-quality, high-throughput, silicon-based DNA synthesis platform, as well as work by Grass and his academic partners in DNA translation and artificial fossils.
In a statement, Twist CEO and co-founder Emily Leproust said: “It’s exciting to ground the fictional series, which expounds beyond the boundaries of what is possible with DNA today, with the reality of preserving ground-breaking cultural media in synthetic DNA. The ability to store digital data in DNA seems futuristic, but the future is now.”
Why store TV shows in DNA?
This act of storing Biohackers in DNA is more than just an advertising ploy; there are real benefits in storing videos, as well as other consumer products like documents and music, in DNA.
Leproust notes: “DNA is an incredible molecule that, by its very nature, provides ultra-high-density storage for thousands of years.” Grass agrees that DNA’s capacity is “many orders of magnitude higher than any other medium”. “Theoretically in a gram of DNA you can pack 200 exabytes of data – that is 200 million terabytes,” he says. “Theoretically, 20 grams of DNA would be enough to store all of the world’s digital data,” Leproust notes.
How well do you really know your competitors?
Access the most comprehensive Company Profiles on the market, powered by GlobalData. Save hours of research. Gain competitive edge.
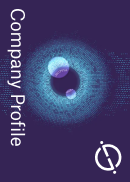
Thank you!
Your download email will arrive shortly
Not ready to buy yet? Download a free sample
We are confident about the unique quality of our Company Profiles. However, we want you to make the most beneficial decision for your business, so we offer a free sample that you can download by submitting the below form
By GlobalDataIn addition, DNA is incredibly stable as a storage medium. “The other media we have, hard drives and SD cards [for example], are only stable for five to 20 years,” notes Grass. “But we have ancient fossil records, which are of years old, and they still contain the natural fossil DNA.” Therefore, “if you want to store things for the future and want to ensure that they survive, DNA is a really good medium to do that,” Grass concludes.
Other advantages of DNA storage highlighted by Leproust include being “relatively cheap to synthesise and copy”, not taking up a lot of physical space, and, finally, its universal format, “which eliminates the evolution of technology advancements that make typical storage media obsolete”. If DNA storage is adopted more widely in the future, it’s unlikely to go the way of the floppy disc.
Practicalities of storing Biohackers in Twist DNA
Fortunately, this useful data storage medium is also relatively straightforward to achieve, as Leproust explains. “First, a data file is converted from its digital sequence of 0s and 1s into a DNA sequence of As, Cs, Ts and Gs; for example, 0 0 = A, 0 1 = C, 1 0 = G and 1 1 = T.” Grass’s collaborator Professor Dr Reinhard Heckel, an assistant professor at the Technical University of Munich, developed a code that makes this translation.
Grass’s lab then adds some error correction algorithms, he explains, to “protect against loss of some info, [for instance] if some of the information degrades or we lose some of it if our reading and writing processes are not perfect”.
Twist then “encodes the DNA data file into short segments of DNA (200 to 300 bases long) that can be synthesized (“written”) and stored”, says Leproust. “In addition to storing part of the data file, each short segment contains an index to indicate its place within the overall data file.”
Grass’s lab then uses its speciality synthetic fossil approach to provide long-term stability for these DNA strands. The team has developed a way to encapsulate the DNA in silica spheres roughly 150 nanometres in diameter. “DNA is only really stable for long-term storage if it’s in a fossil in a matrix, such as in ancient bones,” Grass explains.
Having the first episode of Biohackers stored in DNA is may be useful for its future posterity, but only if it’s easy to convert the DNA code back into a watchable data format.
Grass explains that retrieving the data from the DNA – in a process called ‘reading’ – is done using commercial sequencing technology already being used for genomic sequencing. This decodes the ACTG sequence into the original digital file, as well as applying error correction algorithms.
Broadening the scope of DNA data storage
Leproust is incredibly excited about this project with Netflix and ETH Zurich’s Grass. “This type of high-profile project increases the awareness of the ability to efficiently store information in DNA,” she says.
However, improvements are needed to make DNA storage a reality on a large scale. Although the three components of DNA data storage – DNA writing, storage and reading – have all been demonstrated, the next step is a “race to scale the technologies and, in the process, reduce the cost in order to make a commercially viable product for long-term archival storage”, explains Leproust.
Grass further suggests that wider use of his lab’s error correction algorithms, which are on show in this project, could overcome the current bottleneck in ‘writing’ or synthesising DNA that presently requires perfect sequences, thereby driving the cost down and speeding up the slowest, most expensive part of the process.