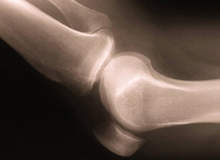
In orthopaedic applications, there is significant need and demand for the development of bone substitutes that are both bioactive and exhibit mechanical properties (both mechanical and surface) comparable to that of natural, healthy bone.
In bone tissue engineering in particular, nano-sized ceramics, polymers, metals and composites thereof may be such materials and have thus been receiving much attention recently. This is because current bone substitutes (or those materials with constituent dimensions greater than 1 micron) have not invoked suitable cellular responses to regenerate enough bone to ensure their success over long periods of time.
Current orthopaedic implant lifetimes are estimated at less than 15 years and have been attributed to the inability of current materials to mimic the dimensions of constituent components of natural bone (such as nanostructured proteins and hydroxyapatite).
For these reasons, nanophase materials (or materials with constituent dimensions less than 100nm) may be a successful alternative to traditional orthopaedic implant materials. The field of nanomedicine has already opened exciting new possibilities for treating bone fractures. Nanotechnology is being used in the next generation of more successful bone implants in order to mimic natural bone.
Nanomaterials offer promising new alternatives since they possess similar dimensions to constituent components of natural tissues, such as proteins and the main inorganic component of bone, hydroxyapatite. It stands to reason that cells in our body are accustomed to nanostructured materials, not conventionally structured, micron implant materials.
NANOPHASE MATERIALS
Only nanotechnology can mimic the natural nanomaterials in our body. In this light, it is widely regarded that surface modification of traditional implant materials (which are smooth at the nanoscale) into biologically inspired nanometre sizes will play a key role in developing the next generation of orthopaedic materials. Similar to creating micron roughness, results of bone formation on implant surfaces with nanometre roughness values seem to be universal (that is, regardless of chemistry) and better than on micron rough surfaces.
How well do you really know your competitors?
Access the most comprehensive Company Profiles on the market, powered by GlobalData. Save hours of research. Gain competitive edge.
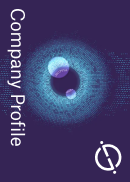
Thank you!
Your download email will arrive shortly
Not ready to buy yet? Download a free sample
We are confident about the unique quality of our Company Profiles. However, we want you to make the most beneficial decision for your business, so we offer a free sample that you can download by submitting the below form
By GlobalDataIn fact, when compared with conventional materials (or materials with micron grain sizes), several studies have reported improved osseo-integration on nanophase implant surfaces created from a wide range of chemistries including ceramics, metals, polymers and composites.
NANOCERAMICS
Increased osteoblast adhesion on nanograined materials was first reported in 1999. Specifically, alumina with grain sizes from 49–67nm and titania with grain sizes from 32–56nm promoted osteoblast adhesion compared to their respective micrograined materials.
Further investigations of these nanoceramics (such as alumina, titania and hydroxyapatite) demonstrated that in vitro osteoblast proliferation and long-term functions (as measured by intra- and extra-cellular matrix protein synthesis such as collagen and alkaline phosphatase, as well as calcium-containing mineral deposition) were enhanced on ceramics with grain or fibre sizes of less than 100nm.
Moreover, osteoblast functions (specifically, viable cell adhesion, proliferation and calcium deposition) were even further increased on nanofibre structures compared to nanospherical structures of alumina. This was believed to occur because, compared to nanospherical geometries, nanofibres more closely approximate the shape of hydroxyapatite crystals and collagen fibres in natural bone.
In addition to osteoblast functions, enhanced osteoclast functions were also observed on nanophase ceramics. For example, osteoclast synthesis of tartrate-resistant acid phosphatase and subsequent formation of resorption pits were up to two times greater on nanophase compared with conventional hydroxyapatite.
Coordinated functions of osteoblasts and osteoclasts are critical for the formation and maintenance of healthy new bone juxtaposed to an orthopaedic implant. Therefore, the results of promoted functions of osteoblasts coupled with greater functions of osteoclasts could assure healthy remodelling of juxtaposed bone formed at implant surfaces composed of nanophase ceramics.
Recent reports have confirmed greater in vivo bone formation next to nano compared with conventional implants. Such results provide promise for the translation of in vitro to in vivo data. Also, decreased functions of competitive cells such as fibroblasts (cells that contribute to fibrous encapsulation and callus formation events that may lead to implant loosening and failure) were observed on nanophase compared to conventional ceramics and polymers.
Specifically, the ratio of osteoblast to fibroblast adhesion increased from 1:1 on conventional alumina to 3:1 on nanophase alumina. Recent studies have also shown that macrophage (a key cell involved in the initial attempt by our body to wall-off foreign implanted materials) functions are significantly decreased on nanostructured compared with currently implanted surfaces.
This shows great versatility in how nanotechnology could improve the success of numerous bone implant chemistries used today.
NANOMETALS AND -POLYMERS
Such trends are not only observed on nanophase ceramics but also on nanometals, nanopolymers and nanocomposites. For example, compared to conventional titanium, titanium alloys (Ti6Al4V) and CoCrMo, respective nanophase metals fabricated by traditional powder metallurgy techniques, increased osteoblast adhesion, proliferation, synthesis of collagen and alkaline phosphatase, as well as deposition of calcium.
For ceramics and metals, most studies conducted to date have created desirable nanometre surface features by decreasing the size of constituent components of materials (such as particles, grains or fibres). However, because of their manufacturing versatility, different techniques have been reported to create nanometre surface features on polymers (such as chemical etching, mould casting, e-beam lithography and polymer demixing; all show promise for orthopaedic applications).
Importantly, increased osteoblast adhesion with decreased fibroblast adhesion was observed on PLGA samples which were cast from nanomaterial compacts compared to conventional compacts. Thus, these proactive nanosurfaces can be copied to polymers to improve their performance as well.
Although increased osteoblast functions and decreased fibroblast functions have been observed on nano compared with conventional compacts, polymers (such as PLGA) may contribute more to rehabilitating damaged bone tissue due to their controllable biodegradability.
That is, while the natural tissue regenerates, these polymers degrade in vivo by hydrolysis into non-toxic, natural metabolites (lactic acid and glycolic acid), which enter into normal metabolic pathways of the tricarboxylic acid cycle to be eventually eliminated from the body in the form of carbon dioxide and water.
COMPOSITES – IMITATING NATURAL BONE
Because natural bone is composed of both organic components (mainly type I collagen) and inorganic components (hydroxyapatite), investigators have also demonstrated enhanced bone cell functions on nanophase ceramic/polymer composites. Specifically, studies conducted to date highlight greater osteoblast responses on composites of PLGA combined separately with nanophase alumina, titania and hydroxyapatite (30:70 ceramic:polymer weight ratio).
For example, up to three times more osteoblasts adhered to PLGA when it contained nanophase compared to conventional titania. However, nanophase ceramic particles tended to agglomerate when added into such polymers.
Therefore, further studies were conducted to increase the dispersion of nanophase titania in PLGA to more effectively promote nano surface roughness; this led to greater osteoblast functions (including adhesion, collagen and alkaline phosphatase synthesis and calcium deposition) on polymer composites with a greater dispersion of nanoceramics.
Increased protein adsorption, important for mediating osteoblast adhesion, was also observed when nano-hydroxyapatite content increased from 10–70%. Moreover, increased mechanical properties (such as compressive modulus) were observed for nanophase hydroxyapatite/poly(lactide) (PLA) composites compared with equivalent micro-sized composites and pure PLA.
Those nano and microscale hydroxyapatite composite scaffolds with high porosity (90% and above) were prepared using thermally induced phase separation techniques. Recently, a novel 3D printing technique has been used to create nanostructured bone substitutes of the exact bone fracture geometry, as determined by CAD.
Besides ceramic/polymer composites, carbon nanofibre/polymer composites have also attracted some attention for orthopaedic applications because of the tailorable electrical and mechanical properties that carbon nanofibres can provide. Promoted responses of osteoblasts have been reported when carbon nanofibres are incorporated into polyurethane (PU).
Specifically, three and four times the number of viable osteoblasts adhered on PU when combined with 10 and 25% carbon nanofibres, respectively. As mentioned, carbon nanofibres promoted osteoblast adhesion in comparison with conventional carbon fibres and titanium (ASTM F-67, Medical Grade 2).
BALANCE OF EVIDENCE
Collectively, these studies exemplify the unprecedented ability of nanophase composites to increase functions of bone cells whether used alone or in polymer composite form. Nanostructured biocomposites provide alternatives not yet fully explored for orthopaedic applications. They may be fabricated to possess similar micro- and nanoarchitecture as that of healthy, physiological bone. Their improved mechanical and biocompatibility properties promise greater orthopaedic implant efficacy in future.
In addition to experimental evidence, there is ample theoretical evidence to support the unique mechanical and surface properties of nanomaterials for tissue engineering applications. For example, mechanical deformation theory indicates that the high-volume fraction of interfacial regions compared to bulk materials leads to increased deformation by grain-boundary sliding and short-range diffusion-healing events as grain size is reduced (thus, increasing ductility for nanocrystalline ceramics).
Also, compared with conventional ceramics, nanophase ceramics possess greater surface roughness, resulting from both decreased grain size and possibly decreased diameter of surface pores. Moreover, nanophase ceramics possess enhanced surface wettability due to greater surface roughness and greater numbers of grain boundaries (or, in other words, material defects) on their surfaces.
Due to such theory, it is clear that the implementation of nanotechnology into tissue engineering does not represent a traditional biomaterial trial-and-error approach, but rather, possesses numerous scientific rationales.
FUTURE PROSPECTS
Despite the challenges that lie ahead, significant evidence now exists elucidating that nanophase materials represent an important growing area of research that may improve bonding between an implant and surrounding bone. By incorporating nanotechnology into orthopaedics, we have learned a tremendous amount of information concerning bone cell recognition with nanostructured surfaces that will certainly aid in improving orthopaedic implant efficacy.