The ubiquitous risk of infection for all types of implanted medical devices has driven a tremendous wave of technological innovation over the past 20 years in the field of anti-microbial coating technology. Nosocomial infections associated with medical implants account for nearly 50% of the estimated two million annual infections in the US, with a total associated treatment cost of over $3bn.
In addition, the related rise in the number of antibiotic-resistant infections, commonly contracted in hospital or clinical settings, has also been a significant impetus for the development of effective and long-lasting antimicrobial coatings.
Several approaches have been employed in the development of anti-microbial coatings. Broadly speaking, they fall into three main categories: anti-adhesives, antibiotics and antiseptics. While each approach has its relative merits and downfalls, it is important to note that all of these methods have generalised benefits with regards to treatment of infection locally, rather than with systemic therapy.
ANTI-ADHESIVES: THE PASSIVE APPROACH
While not strictly anti-microbial, anti-adhesive coatings are nonetheless beneficial for mitigation of device-related infection. Infections develop in an implanted device primarily through the attachment of bacteria, which may already be present in the patient’s skin or body, or introduced to the implant site through the procedure itself. The surface morphology of a device plays an important role in the ability of bacteria to develop attachment points, as the presence of surface pores or microfractures allow bacteria to bind either through electrostatic-driven mechanisms or adhesin excretion.
After attachment, rapid proliferation allows the development of a resident bacterial colony on the device surface, which may in turn create a mucopolysaccharide barrier called a ‘biofilm’. The production of this biofilm allows the colony to resist host immune defenses as well as systemically introduced drug treatments; often, the only effective long-term treatment is removal of the device. Reducing or eliminating the ability of bacteria to colonise the device surface should therefore be a primary goal for designers.
How well do you really know your competitors?
Access the most comprehensive Company Profiles on the market, powered by GlobalData. Save hours of research. Gain competitive edge.
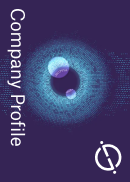
Thank you!
Your download email will arrive shortly
Not ready to buy yet? Download a free sample
We are confident about the unique quality of our Company Profiles. However, we want you to make the most beneficial decision for your business, so we offer a free sample that you can download by submitting the below form
By GlobalDataOne method of reducing the susceptibility of a device to bacterial colonisation is to make the surface hydrophilic, or water-bound, as bacteria are typically better absorbed onto hydrophobic, or non water-bound surfaces. Polymers are particularly suitable as hydrophilic coatings, and some such products have already been commercialised. One product, the Hydrocath catheter (Becton Dickinson), uses a poly-Nvinylpyrrolidone (PVP) coating on a polyurethane base.
The inability of bacteria to adhere to the hydrated surface of this polymer combination has been well-documented. Other polymer surface modifiers include PEG (polyethylene glycol) and PEO (polyethylene oxide).
The main disadvantage of passive anti-adhesive coatings is that their efficacy varies greatly with various bacterial species, and the anti-adhesive properties of these coatings can be severely compromised by conditioning films that develop in vivo. Many bacterial species have receptors that allow them to bind to various beneficial proteins, such as fibronectin, laminin, fibrin and immoglobulins. This effectively allows the colony to establish itself on top of the natural protein layer.
The engineering challenges of developing an anti-adhesive coating that allows just the right interaction between body protein and bacterial attachment are considerable, and such a passive design alone is likely to be insufficient to offer substantial reduction in implant-related infection rates.
ANTI-MICROBIAL COATINGS WITH ANTIBIOTICS
In light of the disadvantages of passive coatings, as well as issues surrounding systemic use of antibiotics, device designers have moved toward integrating active drug delivery at the implant site – an active approach. The key advantage of delivering antibiotics directly at the site of implantation is the high local dosage that can be administered while staying under the systemic toxicity level for that particular drug.
Localised administration also allows for implant area-specific targeting of pathogens, which minimises the risk of side effects or reactions in other areas of the body. Drug delivery through this route also enables the possibility of long-term steady state elution following an initial short-term ‘burst’ of drug activity.
Two approaches have been used to integrate antibiotics into catheter systems. The first involves the production of the antibiotic as an anion, which is then attached to an electrostatically active surface layer containing a cation such as tridodecylmethylammonium chloride (TDMAC).
One product, the BioGuard Spectrum catheter (Cook Critical Care) uses a combination of two antibiotics, minocycline and rifampicin, on both internal and external surfaces using TDMAC. A recent retrospective study indicated that a minocycline/rifampicin-impregnated central venous catheter system was associated with a nine-fold decrease in infection rates.
The second approach involves the direct impregnation of the drug into the polymer matrix before injection moulding or extrusion. Careful engineering design in the choice of materials processing and excipient addition can modify the elution properties of the drug/polymer matrix. Minocycline and rifampicin-treated polyurethane-based catheters have been tested in vivo with significantly reduced infection rates, long anti-microbial activity (three weeks) and long shelf life (one
year).
As device designers are aware, the choice of biocompatible materials for intravascular devices is constrained by requirements of flexibility, surface finish, thrombo-resistance and cost. Despite the challenges involved in the maintenance of these material properties, as well as the new role as an active drug carrier, intravascular devices have been successfully modified to deliver localised antibiotic therapies. Various sidechain functional groups have been introduced into polymer backbones to provide anchor points for antibiotics such as amoxicillin and rifampicin.
Challenges still remain for both approaches to integrating antibiotics into medical devices. From a design perspective, a reasonable drug elution profile may be difficult to achieve for a particular polymer design. In one example, gentamycin delivery from polymethylmethacrylate (PMMA) beads was only 7.5% of the loaded amount, due to the limited ability of the drug to diffuse out of the polymer matrix.
In some instances, designers may therefore feel bound to abandon a well-known, fully characterised material in favour of one more suitable as a carrier for antibiotics. The risk of increased anti-microbial resistance to antibiotics also cannot be ignored, regardless of delivery mechanism. A combinatory approach, involving either multiple antibiotics or an antibiotic / antiseptic combination, is more likely to be successful.
ANTISEPTIC COATINGS
Several non-antibiotic-based coatings with anti-microbial properties have been developed as a response to the difficulties of maintaining sustained anti-microbial activity (greater than one week) and reducing or eliminating the inadvertent development of antibiotic-resistant organisms.
One type is based on the antibacterial properties of silver, which is among the oldest known anti-microbial agents in modern medicine. The key advantages of silver are its remarkably low human toxicity combined with a broad spectrum of antibacterial activity. Silver, in its ionic form, binds to the bacterial DNA, hindering bacterial replication and simultaneous deactivation of metabolic enzymes.
While silver-coated devices such as fixation pins and orthopaedic implants have not been shown to substantially reduce bacterial adhesion, oxidised silver in polymer matrices has exhibited considerable antibacterial activity, acting as reservoirs for the release of silver ions at anti-microbial levels for extended duration (more than three months).
The ability to fine-tune polymer matrix properties by varying melt crystallinity and filler constituents has been shown to modulate silver ion flux, and a multi-layer coating scheme may be used to produce a large initial concentration of silver ions at the implant site, followed by a prolonged steady-state release profile. Silver-based devices that have received US FDA approval include the Bardex I.C. and LubriSil I.C. urinary catheters (C. R. Bard).
Hybrid devices, using a combination of the antiseptic agents chlorhexidine and silver sulfadiazine, have also recently been developed. Chlorhexidine and silver sulfadiazine are believed to have a synergistic effect, as the presence of the chlorhexidine changes the properties of the bacterial membrane, thus allowing silver ions to enter and bind to bacterial DNA.
While several products have been approved, studies comparing these catheters to those containing the antibiotic combination coating of minocycline and rifampicin have shown that the chlorhexidine/silver sulfadiazine catheter system is not as effective at reducing microbial colonisation.
Chlorhexidine has also been the subject of US FDA warnings about patient hypersensitivity, while the increased use of silver itself is causing some concern about the development of silver-resistant bacterial strains.
Other approaches to non-antibiotic coatings include the impregnation of nanosized chitosan particles into materials such as bone cement and the development of coatings that release nitrous oxide (NO). Chitosan, a biopolymer derived from shells of crustaceans, demonstrates low toxicity toward mammalian cells as well as anti-microbial properties, while NO is involved in chemical reactions that produce highly reactive species such as peroxynitrite (-OONO) that have been implicated in bacterial membrane damage.
FUTURE ANTIMICROBIAL ACTIVITY
The research and development efforts for antibacterial coatings have demonstrated substantial progress over the past 20 years, resulting in several approved devices as well as many more in the pipeline. As with many aspects of device development, there is no ‘one size fits all’ approach for the selection of an anti-microbial coating.
A thorough understanding of the target microbial species and an appreciation for potential synergistic combinations of anti-adhesive, antibiotic and antiseptic agents are essential milestones in the development process. Finally, careful consideration of necessary elution or flux profiles will guide material selection and processing, which must, of course, be followed by a rigorous testing regime.