In 1960, scientists Manfred Clynes and Nathan Kline coined the term "cyborg" to describe a symbiosis of man and machine. Since then, the cyborg concept has largely remained the province of sci-fi movies. In the last five or so years, however, the synthesis of man and machine has taken a dramatic step closer to reality, as the work of biomedical engineers, such as Justin C Sanchez, director of the University of Florida (UF) Neuroprosthetics Research Group, demonstrates.
"Many people around the world are trying to alleviate disabilities, or cure diseases of the nervous system," says Sanchez. "There are really two approaches to doing that: a biological approach – such as stem cell research; or what we are trying to do – use engineering principles. So we are trying to design some kind of engineering system that can directly interlink with the nervous system in a functional, meaningful way."
The mission of the Neuroprosthetics Research Group (NRG) is to "develop state-of-the-art novel medical treatments by operating at the interface between basic neural engineering research and clinical care."
This work requires the collaboration of researchers from a number of disciplines. These include computational neuroengineering, microsystems, imaging, materials science and engineering, movement disorder research, integrated circuits research, network-based computing, systems neuroscience, neuropathology, neurosurgery and neurology.
MIND AND BODY
In recent years, a number of scientists have made significant progress toward creating an artificial bridge for communication between the brain and the external world via a computer – a brain-machine interface (BMI).
How well do you really know your competitors?
Access the most comprehensive Company Profiles on the market, powered by GlobalData. Save hours of research. Gain competitive edge.
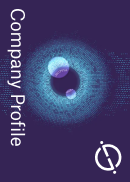
Thank you!
Your download email will arrive shortly
Not ready to buy yet? Download a free sample
We are confident about the unique quality of our Company Profiles. However, we want you to make the most beneficial decision for your business, so we offer a free sample that you can download by submitting the below form
By GlobalDataIn June 2004, for example, a hugely significant event took place in the field of bioengineering and neuroscience when a BMI was implanted into the brain of quadriplegic Matthew Nagle in the US. The procedure was part of a clinical trial pioneered by John Donoghue, professor of neuroscience at Brown University, and the company Cyberkinetics.
The results were impressive. With his brain wired up to a computer, via the BrainGate BMI, Nagle was able to move a cursor, operate a television, and even close the fingers on a prosthetic limb – just by thinking. Although the implant was removed after a year, it was a significant step towards treating diseases of the nervous system via engineering solutions.
This type of research has a variety of applications. "For someone paralysed because of a spinal cord injury, who cannot move their arms and legs, we want to develop a neural interface bypassing that disconnect in the spinal cord, trying to sense and decode information from the brain, allowing that person to communicate directly with a prosthetic limb, a computer – any number of different devices," says Sanchez.
This approach is not just limited to the brain's outputs. "Take a different situation like epilepsy; there we want to interact with abnormally firing neurons, stimulating the brain and putting information back into the brain telling those neurons to stop firing in that abnormal way." While each approach offers many new treatment possibilities, technology is serving as the enabler.
Donoghue's work on understanding interneuron communication; Richard Normann's invention of the Utah electrode array for implanting silicon microelectrodes into the brain; Miguel Nicolelis getting monkeys to move on-screen cursors through thought alone; these have all been important milestones on the road to creating a commercial BMI.
Now Sanchez and the research team at UF, consisting of experts from UF's colleges of medicine and engineering and the McKnight Brain Institute, are attempting to move the BMI a step further, creating a wireless solution in conjunction with a miniaturised implantable device.
Sanchez outlines the basic challenge: "A person intends to move, and some neurons in their motor system start to fire. You need an electrode to sense this activity. The electrode sends this signal to an amplifier bringing up the level of that signal. The telemetry system wirelessly transmits the information to a digital signal device. That device decodes the information and decides what the person intends to do. Finally, a command signal is sent to a prosthetic device, and from that
device feedback returned to the patient."
Where other researchers have used the Utah electrode array – which looks like a miniature bed of nails – in conjunction with a wired device, Sanchez and colleagues are adopting a different approach.
"We want to build a flexible electrode array using a polyamide substrate, and within that substrate, patterning traces of metal which become the electrodes and the routing of the circuitry for the amplification and wireless system," he says. "So there are several components: the flexible substrate made of metal and polyamide, and attached on top of that substrate, customised hardware and electronics for the amplifier and wireless system."
All of this technology goes into an implantable neuroprosthetic chip the size of a US quarter.
"The signals we are trying to sense from the brain are in the order of micro volts," says Sanchez. "We have to amplify them in a meaningful way, then wirelessly transmit those signals outside of the skull. So we envision this device sitting underneath the scalp, with the electrodes going through the skull and into the brain, and then wirelessly transmitting all this information out. Potentially, several of these chips could be placed in strategic parts of the brain that
control a variety of functions."
THE CHALLENGES AHEAD
If the essential concept sounds simple, the practical challenge is not. Extracting and decoding signals from a fraction of the 100 billion neurons that make up a person's 3lb (on average) of brain tissue, and then doing something meaningful with that information, is a task that tests the limits of existing technologies and our knowledge about the brain.
One problem is scale. "Many clinical devices out at the moment are using large electrodes to sense brain rhythms, so you can't particularly determine the information from individual neurons," says Sanchez. "As we study these systems more, we find that going to the micro level of the individual neurons gives the most natural and precise information about what a person is intending to do, or what abnormal neurons are actually doing." Current technologies only allow
researchers to interface with hundreds of neurons at a time, but the most effective interfaces will probably have to deal with thousands of neurons to capture all of the intricate complexities in the activities of daily life.
"The more neurons you have the greater probability of finding the relevant neurons essential for neural decoding or neural interfacing. But to do thousands of neurons requires another technology leap to enable us to process and transmit all that information simultaneously," says Sanchez.
Another issue is bandwidth. The amount of information being picked up and transmitted is enormous. Various strategies are employed to reduce the amount of information being sent down the 'pipe'; one promising solution the team is working on is to use new ways of encoding the waveforms and then send out pulse-based representations of them.
Sanchez must also deal with the fleeting timescales involved. "You need to deliver the relevant information in a meaningful timescale for the individual," he says. "Think about moving and you have tens of milliseconds to see the result of your thoughts. Work with a timeframe any longer than that and you will notice some delay. That makes your ability to interact with systems very difficult.
"If we sense someone's neural processes, we need to decode that information in very short timescales. So every 50 milliseconds we want to make some kind of decision. In that timeframe (or smaller) we have to transmit and decode the information, and use the information to control something."
To decode the information the UF researchers need to extract clinically significant information from the signals produced by the brain – biosignal processing. Here they will also need to develop innovative solutions, as existing electrical engineering techniques to handle man-made signals do not always cope well with signals generated by the nervous system.
ENGINEERING THE DEVICE
Once researchers can understand and decode the information, the technical challenge is to bring that information to a small implantable device; this requires significant engineering advancement. Currently there are two main strategies adopted in building neural interfaces. One is to do all the neural decoding directly on the implantable chip and then send out results. Some people believe, however, that the all-in-one solution involves too much power and computational complexity. An
alternative is to send out the information to a small external device that can do the neural decoding.
"With current technology it makes most sense to have the implantable chip on the brain use wireless telemetry to send information to some sort of handheld device collecting the transmitted information," says Sanchez.
Boosting the signals from the brain requires an onboard amplifier, and this in turn demands a power source. So Sanchez and the team at UF are also designing a miniature rechargeable lithium-ion battery that can be recharged externally through the skin. Plus, if you implant something into a person there is always a risk that it will be rejected; yet another issue that the UF researchers must contend with.
"We want to bring these devices to the clinical realm," says Sanchez. "If we implant something in the brain we would like it to be functional throughout a person's lifetime. But with anything implanted in your body, there is an issue with the reactivity of the body to that man-made device. The same holds true for electrodes implanted in the brain: the body responds to them and it affects the ability for those electrodes to sense the relevant signals.
"Several approaches can be used to try to make these things more biocompatible and viable for long periods of time. You can coat them with a material, for example, or make them smaller so that they are kind of 'stealth' electrodes. "
The firing of neurons may be measured in milliseconds, but fortunately for Sanchez and the rest of the team, the terms of a $1.6m grant from the National Institutes of Health allow them a little longer to complete their research. The target is to get the device fully parameterised, with multiple prototypes, and functional within four years.
The work will require a multi-disciplinary effort. The UF team includes professors with expertise in telemetry (Rizwan Bashirullah, PhD), amplification (John G Harris, PhD), microfabrication (Toshi Nishida, PhD), signal processing (Jose C Principe, PhD) and computing (Jose Fortes, PhD). Sanchez works in paediatrics, neuroscience and biomedical engineering.
But the work is not just cutting-edge science; it is a triumph of the imagination. If successful, Sanchez and the UF team will bring a barely hoped-for freedom to spinal injury patients, and the promise of treatment for other brain mediated diseases of the motor system such as Parkinson's disease, as well as epilepsy and similar brain disorders.
Ironically, the results of such a major breakthrough in biomedical engineering would almost certainly go unnoticed by all but a few. "What is the ultimate device?" says Sanchez. "You would like to see a person on the street and while their clinician knows they are disabled, no one else would be able tell that they have an implantable device that is doing some sort of neural processing, enabling them to overcome their disabilities and enjoy new forms of communication and
control."