One of the great advantages of the development of minimally invasive or miniaturised medical technology products is a greatly reduced impact on the patient. With minimally invasive techniques, or miniature implants, there can be a massive reduction in the trauma of surgery and greatly accelerated recovery times leading, ultimately, to a much faster return to an active role in society of the patient and often reduced hospital costs.
Miniaturisation of medical technology products has been a goal for many years and much has been achieved via the traditional ‘engineering’ route. However, with the advent of nanotechnology and other new disciplines, the emphasis is gradually changing, from a ‘top down’ engineering approach to a ‘bottom up’ approach where structures are assembled from more basic materials and components. This is mirroring natural biological approaches, the essential difference being that researchers and, increasingly, manufacturers now have the ability to manipulate materials at the nanoscale (between 1nm and 100nm).
One of the most interesting characteristics of current medical technology innovation is the convergent nature of new products. Increasingly, innovation may marry new materials with improved imaging techniques, telematics, cell biology, miniaturisation and multifunctionality, such as the combination of diagnosis and treatment, often facilitated by the application of nanotechnology.
DIAGNOSTIC DEVICES
Applications of this approach are already being developed in miniaturised diagnostic devices. For example, microfabricated cantilever sensors are being used as ultrasensitive mechanical sensors converting (bio)chemical or physical processes into a recordable signal in microelectromechanical systems (MEMS) or nanoelectromechanical systems (NEMS).
One specific application currently being evaluated for cantilever-based sensors is for the early diagnosis of diabetes mellitus where the presence of a diagnostic ketone, acetone, could be detected in a patient’s breath in tiny amounts. Further applications predicted for nano-cantilever arrays include the early detection of cancer-associated molecules.
How well do you really know your competitors?
Access the most comprehensive Company Profiles on the market, powered by GlobalData. Save hours of research. Gain competitive edge.
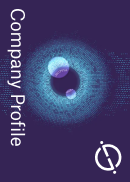
Thank you!
Your download email will arrive shortly
Not ready to buy yet? Download a free sample
We are confident about the unique quality of our Company Profiles. However, we want you to make the most beneficial decision for your business, so we offer a free sample that you can download by submitting the below form
By GlobalDataIt is, indeed, the fact that only nano- or pico-litres of the substance to be measured are needed in such nanotech-engineered diagnostic devices that makes them so useful. Earlier diagnosis will be facilitated, and it may even become possible to detect marker molecules at the cellular level.
Further examples of promising areas of research in relation to nanotechnology and diagnostic devices include the use of:
- Carbon nanotubes, which can be used as amperometric or fluorescence biosensors, e.g. for the detection of glucose in blood or urine, or for the detection of CO2 in capnography.
- Nanowires, for the biodetection of viruses or of various biomolecules.
- Dendrimers, which can be used to encapsulate ‘guest molecules’ to diagnose heart muscle damage or provide improved contrast media for magnetic resonance imaging.
- ‘Quantum dots’, which can be used in high-resolution optical imaging.
Furthermore, some of these new materials, such as dendrimers and nanocapsules, offer the possibility for a new type of therapy, sometimes referred to as ‘theranostics’, that combines the ability to image the movement of the nanoparticles, often associated with a lymphocyte or an antibody to a target site such as a tumour, with the activation of a ‘payload’ such as a drug or semi-metallic low-power radiation emitter capable of being activated at the desired local site.
INTEGRATION OF MEMS AND NEMS INTO PRODUCTS
There has been considerable research aimed at incorporating MEMS into medical technology. At the same time, MEMS themselves are shrinking towards the nanoscale to become NEMS.
MEMs are already widely used in medical technology, a common example being piezo-resistive silicon pressure microsensors as used in applications such as blood pressure and respiratory monitors, kidney dialysis machines and hearing aids. A more unusual application is in the so-called ‘data knife’ where pressure sensors are built into an ultrasonic scalpel used for very exacting procedures such as brain surgery. A related device is the MEMs accelerometer, widely used in implantable cardiac pacemakers, where the device detects patient exertion levels and adjusts the output signal accordingly.
Further examples of medical technologies under development where MEMs are currently being applied are:
- Subretinal and epiretinal implants, where artificial devices are being interfaced with the existing retinal neural cells.
- Artificial synapse chips (also being considered for visual rehabilitation).
- ‘Spintronic’ devices, where the effect is due to the spin of electrons rather than their charge enabling further miniaturisation and power enhancement. These are likely to have an impact in implantable cardiac devices and in cochlear implants.
In all of these areas, further size and power consumption reductions are likely as MEMS give way to NEM where biological and electromechanical systems integrate at a cellular level. It has already been demonstrated that nerve cells can grow on silicon-based substrates, thereby opening up the possibility of a new generation of novel biomechanical devices.
REGULATORY ISSUES
While some applications of NEMS and MEMS have already reached the market, there are many others in the pipeline. However, several key issues need to be addressed to bring innovative medical technologies to the patient.
The convergence of differing technologies and disciplines that characterises much medical innovation brings with it regulatory challenges. Some new medical technologies cross ‘traditional’ demarcation boundaries between different regulatory systems, although it is likely that those described above will fall within the scope of existing European medical device directives. However, the existing directives were not conceived with some of these novel technologies in mind and it is likely that some aspects will need to be reviewed.
The first steps were taken on 5 July 2005 by European regulators to address this issue, with the first meeting of a new Working Group on new and emerging technologies established under the Medical Device Experts Group of the Medical Device Directive, following a successful workshop between industry and regulators on the same topic. In addition, further guidance or other measures will probably be necessary to address specific demarcation issues between different European Directives and Regulations.
HEALTHCARE TECHNOLOGY ASSESSMENT
Healthcare technology assessment (HTA) is a tool increasingly used by national authorities to assess the value of new medical technologies. The intention of HTA is to apply systematic methods of scientific inquiry to the evaluation and use of new or existing healthcare technologies. This evaluation can focus on all impacts of the respective healthcare technology, ranging from its ethical to its economical implications.
The overall objective of any HTA is to provide solid and objective grounds for decision-making on the provision and the funding of healthcare. HTA is rapidly increasing in importance as EU member states seek to apply their limited healthcare budgets more effectively.
A key need will be to teach HTA assessors and those who base decisions on HTA about innovations based on nanotechnology. This is particularly important, as HTA has been traditionally applied to pharmaceutical products and a transposition of the methodology to nanotech-based and other innovative medical technology products and the interpretation of the results will require appropriate adaptation.
One particular challenge for novel products is to demonstrate to authorities that what may appear to be a more expensive product or treatment may, in fact, provide much improved patient recovery times, reduced hospital stays and a faster return to active life in the community of a patient, possibly greatly reducing longer-term costs.
RISK ISSUES
As with all medical technologies, the use of nanotechnology needs to be accompanied by a systematic approach to risk analysis and risk management together with a clear and unambiguous communication of both the benefits of the therapy and of any residual risks that remain. This approach is already the norm for medical devices and is a part of the medical device directives. Particular attention, however, needs to be paid to nanotechnology-based products, and particularly to clear communication of their risks and benefits, because of the high public profile nanotechnology has been accorded in the media.
PROFESSIONAL UPTAKE OF NEW TECHNOLOGY
Medical professional attitudes toward the adoption of new medical technologies can vary widely from country to country due to cultural differences, training and local practice. New medical technologies and techniques may also affect working practices. Effective communication therefore needs to take place between researchers, manufacturers and medical professionals in introducing novel products and a clear emphasis placed on benefits to the patient that may result.
REIMBURSEMENT
Reimbursement of medical technology products is a familiar issue for all manufacturers and is compounded by the fact that reimbursement systems vary widely from country to country, including in relation to the reimbursement of new technology products. In many cases it is linked to HTA assessment. While it is beyond the scope of this article to address reimbursement issues, the attention of researchers and manufacturers is drawn to the fact that eventual reimbursement of new technology products is an extremely important factor, particularly in view of the costs of research and development.