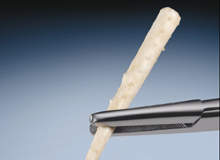
Replacing diseased or damaged tissues with synthetic prostheses or donated organs has been the primary mode of restoration of function in a clinical setting. However, over the past decade, a new approach based on tissue engineering for the in vitro formation of functional tissues for use in vivo has emerged as an alternative solution to a growing problem.
Medical devices, particularly those based on tissue engineering, require a biomaterial scaffold as a critical component of the system, cells (often stem cells) and a bioreactor to provide an environment with appropriate signals to the cells (chemical, biochemical, mechanical and/or electrical, depending on the nature of the tissue to be grown). The scaffolds provide a temporary structure upon which cells adhere, migrate, replicate and differentiate into new tissue structures (extracellular matrix) over time. Thus, the biomaterial scaffold is at the centre of any successful tissue engineering strategy and provides many essential features and cues to direct the cells toward a functional outcome.
Some of these functions include:
- Surface morphology and chemistry: to support cell adhesion, spreading and differentiation to improve biocompatibility and maintain suitable osmotic balance.
- Mechanical properties: to provide mechanical support during new tissue formation in vitro and in vivo and provide mechanical signalling to cells in vitro and in vivo to optimise the structural and mechanical functions of the new extracellular matrix being formed.
- Degradablity: degradation at a rate that is commensurate with new tissue formation in vitro and in vivo to maintain function during the transition from the laboratory to a clinically relevant implant.
Based on these functions for biomaterial scaffolds, a range of polymers are often used in the field of tissue engineering, depending on the tissue or device. Biopolymer-based scaffolds are particularly applicable for these needs due to their biocompatibility, versatility in terms of processing and structural outcomes, impressive range of mechanical properties, controllable rates of degradation and diversity in chemistry – to facilitate cross-linking or coupling of cell-specific factors.
The extracellular matrix forms the architectural and functional basis for all tissues and organs in the body, including biological signalling and biomechanics. These matrices are characterised by a complex structural hierarchy of biopolymers in 3D architectures. Therefore, these types of biopolymers have become logical targets in many studies with polymeric biomaterial scaffolds for tissue engineering. Specifically, these types of biopolymers offer:
- A range of mechanical properties. They range from the strongest natural fibres known, in the case of silk protein fibres, to gels of polysaccharides and flexible films from collagens.
- Processability. They can be transformed into a range of material formats through solution processing and, in some cases, more traditional plastic processing based on thermal methods.
- Chemical modification. The diversity and accessability of surface chemistry offers relatively simple options for surface ‘decoration’, such as with cell binding domains (arginine-glycine-aspartic acid peptide sequences), cytokines (protein-based cell signalling factors) or laminates with other biopolymers to form composite structures. The process can usually be carried out with carbodiimide chemistry, often resulting in the retention of biological function of even labile molecules such as cytokines. Cross-linking using the above chemistry or via biocatalysis with transglutamidases and peroxidases can also be utilised.
- Degradability. Rates of degradation range from very slow to very fast, and these rates can be controlled based on the biopolymer used, the processing mode to influence bulk structure and surface area, and surface chemical modifications. Furthermore, the degradation products from these biopolymers are the normal metabolic building blocks found in cells.
- Tailorability. Advances in genetic engineering techniques provide significant control over sequence chemistry and polymer molecular weight through recombinant DNA techniques.
Commonly used synthetic polymers, often studied as biomaterials for tissue engineering scaffolds, cannot offer the above combination of features. Furthermore, metals and ceramics are rarely used as scaffolds for tissue engineering due to their lack of biodegradability and mechanical mismatches with tissues. While these biomaterials are important in many biomedical devices, the biological, chemical and physical requirements demanded in tissue engineering dictate a major role for biopolymers.
How well do you really know your competitors?
Access the most comprehensive Company Profiles on the market, powered by GlobalData. Save hours of research. Gain competitive edge.
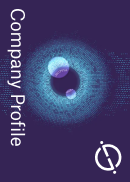
Thank you!
Your download email will arrive shortly
Not ready to buy yet? Download a free sample
We are confident about the unique quality of our Company Profiles. However, we want you to make the most beneficial decision for your business, so we offer a free sample that you can download by submitting the below form
By GlobalDataBIOPOLYMERIC MATERIALS
Of the biopolymers commonly studied for polymeric scaffolds in the field of tissue engineering, polysaccharides are of particular interest in joint-related repairs based on their role as glycosaminoglycans in mammalian extracellular matrices. Hyaluronate and alginates are the most intensively studied, due to their ease of processing into useful structures. The hydrophilic nature of polysaccharides permits hydrogel formation, which is often useful for cell encapsulation. These polymers exhibit low crystallinity and minimal inflammation response.
Scaffolds formed from proteins are particularly suited for tissue engineering as they comprise the structural basis for the extracellular matrices of tissues. Fibrous proteins (collagens, silks) in particular are attractive, due to their architectural features and impressive mechanical properties. These materials are often studied as porous scaffolds for orthopaedic tissue engineering, as well as in fibre forms for ligaments and tendons. In some cases, such as with collagens, concerns over bioburdens remain an issue when bovine sources of the material are utilised. There are no similar concerns with silks and elastins.
Polyhydroxyalkanoates, polyesters comprised of hydroxyl fatty acid monomers, are normally produced as granules in some bacteria for intracellular storage. Depending on the structure, these polymers are thermoplastic and can be melt processed, injection moulded or solution cast – thus they can be handled more like commodity plastics.
This is not the case with the proteins and polysaccharides which are most often processed via solution methods, as they tend to degrade prior to melting due to the extensive hydrogen bonding characteristic of many of these biopolymers. The elastins are an exception as they undergo inverse thermal transitions which provide novel opportunities in biomaterial behaviour in tissue engineering applications.
Many of these biopolymers have been successfully processed and used in various fibre forms, using standard solvent extrusion spinning methods or via electrospinning to form nano-scale diameter fibres. Porous 3D spongy matrices are formed via lyophilisation, salt leaching or gas evolution methods. Films and hydrogels are also common products used in scaffolds. Efforts are also underway in many laboratories to improve controlled premineralisation of some of these biopolymer matrices as a way to stiffen the materials to improve their mechanical properties for some tissue engineering applications.
TISSUE ENGINEERING
Polysaccharide hydrogel scaffolds are commonly studied for engineering replacement connective tissues. Cartilage, due to its avascular nature, low metabolic requirements, limited capacity for self-repair and importance in degenerative diseases such as arthritis is a common target for tissue engineering. The biochemical similarity of polysaccharide hydrogels with natural glycosaminoglycans found in this tissue is a rationale for the use of polysaccharides as scaffolds for tissue engineering cartilage. This rationale also applies to chitosan when combined with its ability to stimulate chondrogenesis.
Alginate has been intensively studied for use in cartilage tissue engineering due to its bioinductive capacity with chondrocytes and ease of moulding into suitable morphologies for joint replacement. Hyaluronic acid is found in cartilage, and its viscoelastic properties result in its use for the repair of damaged cartilage tissue. The hydrated state of the polymer reduces cell adhesion and can often result in cell loss during fluid flow in bioreactors used for studies in tissue engineering.
This problem can be addressed through chemical modification of the biopolymer. Collagens and silks have also been used as biomaterial scaffolds in cartilage tissue engineering and offer benefits in terms of mechanical properties when compared with the hydrogel polysaccharide systems.
Collagen and silk-based biomaterials for ligament tissue engineering have been studied. The slow degradation of silk combined with the unique combination of mechanical properties has provided improved outcomes for the silk-based systems. Matrices in fibre forms, emulating textile engineering approaches and studied in a dynamic mechanical environment in combination with stem cells, have resulted in functional ligament tissue outcomes in vitro.
Polysaccharide hydrogels, despite their relatively weak mechanical properties, have demonstrated applicability as scaffolds with osteoprogenitor cells for the repair of bone defects in non-weight-bearing areas. Collagen and silk porous sponge matrices are actively studied for bone tissue engineering as well, with control of pore size to optimise transport, in a range of osteogenic outcomes.
Additional options for these matrices include carriers for cytokines and conduits to optimise fluid flow. Recent studies with silk-based biomaterials provide mineralisation templating effects that offer unique features in the process, starting with stem cells with osteogenic stimulants and/or mechanical compression. These slowly degrading biopolymer matrices offer a unique option for the control of mineralisation morphology.
Blood vessel tissue engineering has utilised bovine collagen as a scaffold for remodelling into functional blood vessels. Elastin-based materials are also being actively studied toward this goal.