
Scientists across many disciplines are working together with materials at nanometre sizes to find exciting applications in electronics, life sciences, chemistry and materials. The uses across different sectors vary enormously, but in the medical industry their use is now of vital importance.
In materials, there are applications in making clothes stain and water resistant. Advances have also been made in producing extremely light and strong metals. Recently, a bike made from carbon nanotubes was so light that weights had to be added to allow it to compete professionally.
Now experts are even making materials that adapt to their surroundings, such as car bodies that change their properties on impact to absorb the effects of a crash. The motor industry is also being aided by nanocrystals which act as fade and scratch-resistant paint surfaces, and nanoparticles that increase battery capacity.
Most of this work, however, is still only in the laboratory. It could be another ten to 15 years before much of it starts to work its way into commercial and industrial products.
In the medical field, there is work in DNA fingerprints (a DNA strand is ten times wider than a 1nm nanotube), drug delivery and disease detection.
“What we have done on the nanotechnology front is identify applications in the material sciences, of which some are in the life sciences and medical areas,” says Grant Drenkow, nanotechnology programme manager at Agilent Technologies. “There are two or three areas that could benefit the medical sector.”
How well do you really know your competitors?
Access the most comprehensive Company Profiles on the market, powered by GlobalData. Save hours of research. Gain competitive edge.
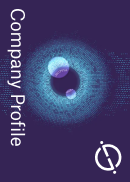
Thank you!
Your download email will arrive shortly
Not ready to buy yet? Download a free sample
We are confident about the unique quality of our Company Profiles. However, we want you to make the most beneficial decision for your business, so we offer a free sample that you can download by submitting the below form
By GlobalDataTheory in practice
A big boost to such work is the atomic force microscope. This allows scientists to look at individual cells down to the atomic scale, which then lets them study DNA strands. The microscope can also probe inside fluids.
“We are imaging the components within the cell,” says Drenkow. “We can look at the different molecules, at the RNA, DNA and so on.”
This technology even allows the scientists to drag a probe across the surface of the cell and create a three-dimensional image of the structure. Although this is still being used in research laboratories rather than hospitals, it is giving biophysicists a greater insight into what is happening at cellular level.
More recently, this work has been improved with the addition of digital microscopy, also known as optical fluorescent microscopy. With a normal optical microscope, a scientist can look close up at what is happening on a slide illuminated by a light source. The optical fluorescent microscope is octagonal with each of the eight sides having its own light sources and multiple digital video cameras. Mirrors and filters help to focus on the sample, which sits in an environmental chamber within the microscope. The multiple cameras feed live images of the cells to a computer where they can be studied in real time and from different aspects.
“You can look at different interactions within the cell simultaneously and you can do everything directly on the computer screen,” says Drenkow.
Another type of instrument that is impacting the medical field is the particle analyser. First, it can be used in the design and manufacture of drugs. The size of the particles that make up the drugs can influence the delivery of the drug to a specific part of the body. This is particularly important with young children where there is risk of clogging up capillaries if the particles are too large. “You need to be really careful to get the right size,” says Drenkow. “Particles are becoming smaller so that you can deliver them more accurately around the body.”
Research is also looking at using these particles to penetrate cell membranes and thus deliver the drugs direct to the cell cytoplasm. This is important because it can act directly on where some diseases are operating.
A second use of a particle analyser is to put an electrical charge on the particle, what is known as the zeta potential. If the drug is dissolved in a fluid, the particles need to repel each other and not stick together to form lumps, which is extremely important if the drug is administered intravenously. The zeta potential achieves this. In another life this technology has also been taken up by beverage companies to help instant drink mixes dissolve faster.
Academic interests
Another body looking at nanoparticles for medical applications is the University of Urbin in Italy. In its research, funded partly by Philips Research, the university is trying to encapsulate magnetic nanoparticles inside living blood cells. This will make the blood cells more visible for longer periods of time when used with medical imaging equipment. Existing contrast agents are quickly excreted from the blood via the liver, making it difficult to obtain accurate images.
The new particles that the university has developed go right inside the red blood cells and can last up to 120 days, often longer than the life of the blood cell itself.
Philips and the university have entered into a two-and-a-half-year research project to investigate these particles further. The goal is to find a better way of treating cardiovascular diseases. Medical imaging is used in this area to help guide surgeons using a catheter inserted into the heart to destroy tissue causing abnormal electrical signals.
This complex procedure can take hours which stretches the use of normal contrast agents to highlight blood flow. The new nanoparticles could, in future, help the surgeon see the volume of blood in each of the heart’s chambers.
This process could also be used as another drug delivery method as it has the potential keep the drug in the body longer. This would aid some treatments where the medicines effectiveness is limited because the body expels them too quickly.
In Texas, scientists at Rice University in Houston have been working on producing pure gold nanorods. These are wand-like nanoparticles that could help in diagnosing diseases.
The university is also looking at using nanoparticles coated with gold for cancer treatments.
Scientists have found already that these can kill cancer tumours in mice, using an infra-red laser to heat up the gold and kill the cancer cells to which it is attached.
Throughout all of the research being undertaken today, what is clear is that it is bringing together scientists from all over the world and from a wide circle of disciplines. While the eventual uses of the technology might not be so well defined, many feel that impressive as recent achievements are, what is most exciting is that we have only just begun to scratch the surface of all this technology has to offer.