
Imagine a medical device that could be worn, like skin, and transmit real-time biological information for remote monitoring, drug administration or even to mimic neural pathways between the brain and prosthetic limbs. This may sound like science fiction, but Dr Stephanie Lacour, principal investigator, engineering research fellow at Cambridge University, and a team of UK researchers have embarked on an ambitious project that could allow medical devices to be connected through RF antennas, worn on areas of the body never before thought possible.
Lacour has been working on conformable electronics since 2001, and specifically for biomedical applications since 2005. Her group has been developing novel approaches to engineer stretchable bio-electronic systems based on the integration of metal films, semiconductor circuits and transducers on ultra-compliant substrates, with a special emphasis on matching the biomechanical interface of cell/biological tissue and devices.
Though the elastic antennas and RF components project has been running for only a few months, the groundwork for this study has been in the making for a few years. In an article co-authored by Lacour, then at Princeton University, in August 2005, “Stretchable Interconnects for Elastic Electronic Surfaces”, the team was able to demonstrate the potential feasibility of using compliant polymer substrates to hold sub-circuit islands, interconnected with stretchable strips of thin gold film. This project produced the first elastic circuit, made of transistors that were able to maintain their electrical conduction, even when stretched and relaxed by 12% strain.
Following on from this success, the team developed a stretchable microelectrode array (SMEA) in 2006 that could continuously record neuronal function, pre, and post brain stretch injury. The device was fabricated on a “polydimethylsiloxane (PDMS) substrate with stretchable, 100μm-wide, 25nm-thick gold electrodes”.
The SMEA design has since been further improved by the team. The outcome of that project showed promising results and a definitive improvement over existing MCS arrays.
“I’m particularly interested in making neural implants,” says Lacour. “We have a project here at Cambridge University where we are looking into fabricating peripheral nerve implants using soft polymers and embedded electrodes so that we can record neuronal activity in vivo from peripheral nerves. The project is across the University of Cambridge, University of Birmingham and King’s College London.”
How well do you really know your competitors?
Access the most comprehensive Company Profiles on the market, powered by GlobalData. Save hours of research. Gain competitive edge.
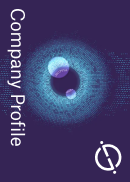
Thank you!
Your download email will arrive shortly
Not ready to buy yet? Download a free sample
We are confident about the unique quality of our Company Profiles. However, we want you to make the most beneficial decision for your business, so we offer a free sample that you can download by submitting the below form
By GlobalDataThe new project on compliant RF components is just starting. Lacour is quick to point out that it will be at least six to 12 months before any definitive data from the project can be exploited and therefore discussions at this point remain speculative. Though still in its infancy, there are high expectations that the elastic antennas and RF components project could revolutionise the medical device industry over the next decade.
Theoretically, these circuits could be designed to fit a peripheral nerve in vivo or be applied to patches of skin. The potential applications for these elastic circuits are many. Medical devices, novel approaches to tissue repair and drug delivery platforms could all directly benefit from a successful outcome on the new RF antenna project. But the consumer electronics market stands to benefit sooner if the project succeeds, with hand-held devices, robotics and even the arts and
entertainment industry being likely candidates for future development.
Joint effort
The project, a collaborative effort between the University of Cambridge engineering department and the University of Sheffield Electrical Engineering Department, is being funded by the Engineering and Physical Sciences Research Council. In terms of how the project will be maintained across two universities, Lacour states that the two groups have complementary areas of expertise and will be working directly together to create and test prototypes
“In Cambridge, we are in charge of making the devices,” she explains. “The Sheffield team are experts in RF component characterisations, so they will conduct the electrical characterisations of the devices we’re making here.
“Once the preliminary set up and training period is complete, the two teams will further join efforts to do the electromechanical characterisation of the compliant electrical devices. In particular we will look at how the antennas will respond to stretch. Here in Cambridge we have a set of customised tools that can be used to manipulate these devices in one direction or multiple directions, simultaneously. Sheffield will use their equipment to measure the antenna response under mechanical stress.”
Until now, the electrical engineers have been working on silicon chips, like those to be found on computers or mobile phones; and these are pretty rigid. The electronics industry has been designed to process >10in-diameter silicon wafers or even extremely large area glass plates, but those integrated circuits are made flat, on a 2D plane.
“If you look at our bodies, we are in three dimensions and we’re moving,” Lacour adds. “When it comes to interfacing electronics with the body, you hit a mechanical mismatch between the hard planar electronic devices with the soft, wet and moving environment of biological tissue.”
Multi-material compliance
The project team is trying to implement the function of electronic devices onto materials which are extremely compliant and would behave mechanically similarly to biological tissues. “The substrates I am primarily using are rubbery materials called elastomers,” Lacour explains. “They behave mechanically like a rubber band, so we are trying to prepare devices directly onto these rubbery materials. We are at the stage of evaluating what type of electronic components we can fabricate on this soft material and antennas are one of the devices we’re exploring.”
“Whether electronic components are implanted in the body or grafted onto the skin, they will require power and signal transmission to another remote electronic component such as a hand held device or laptop,” she explains. “With the antenna project, we want to explore if we can build such devices on soft materials, and how they respond to mechanical deformation. In bioelectronics, you are really thinking about miniaturised elements and electronic components that interfere “mechanically” very little, or not at all, with biological tissues.
“The antennas would permit the RF link between the implanted devices or sensors to the off-body devices. But first we need to evaluate what type of signals ultra-compliant RF components can transmit and receive.” Currently, there are flexible antennas available on the consumer market in the form of RFID tags. These tags allow deformation of the antenna on a two-dimensional plane in terms of bending, folding or rolling and are widely used in the clothing market. However, they are not designed to be stretched without losing their electronic function and signal strength.
“Our project is exploring the feasibility of making antennas that could not only cover large areas of the body but also joints,” says Lacour. “For example, if the patient were to bend their finger or elbow, if the antenna has to be placed at the joint, then it can accommodate the deformation without impairing its electrical response. So that is where we are bringing the mechanical compliance with the electronic functionality.”
Biological focus
“The substrates we are working with are silicones that exist in different grades – medical and non-medical,” explains Lacour. “At the moment, because non-medical grades are cheaper, we are working with these. But because the medical grade substrates do exist, those substrates would be biocompatible. Then we would have to evaluate biocompatibility and toxicity in vitro and in vivo of the complete components. However, this is beyond the scope of the current project.”
The project is focusing on evaluating the feasibility of the deformable antennas and measuring their electrical response when they are flat and relaxed and when they are stretched. Once the team has established these characteristics, then it will think further about how to integrate them into real biological applications.”
Potential uses for the ultra-compliant antennas could include biosensors used to monitor glucose, or connecting to neural implants that transmit neuron pulses (neuronal signals). But the full scope of applications will not be clear until the project has determined feasibilities.
In terms of timescale, Lacour believes that an external version of these antennas could reach the market within five years of the project’s end date. “There are different levels of biocompatibility. If you are talking about integrating antennas into clothes that are worn on the body, I think if we are successful in this project, we might have some prototypes in the lab by the end of the project; for example, patched somewhere onto a piece of a t-shirt. Putting the devices onto something that would be worn could be a five-year timescale. If we then want to move onto skin or implantable devices, it would be much longer-term.”