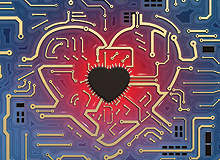
Miniscule implantable medical devices powered by the heartbeat, the flow of blood or even the breathing of a patient might sound far-fetched, but groundbreaking research in the emerging field of ‘nanopiezotronics’ is rapidly making such seemingly outlandish technology a distinct possibility.
Nanopiezotronics is technology that could, in time, transform not only how medical devices are powered, but also the entire medical device industry.
“It is true that, to a lot of people, what we are doing looks and sounds a bit like science fiction,” says Dr Zhong Lin Wang, director of the Center for Nanostructure Characterization at the Georgia Institute of Technology in Atlanta, one of the leading pioneers in this field, and the man who even coined the term nanopiezotronics.
“It has been a slow evolution, with a lot of work over the past six years, and it is now that things are really starting to happen. We are much more confident that we are closer to practical applications than we were even two or three years ago.
“To understand nanopiezotronics it is first necessary to understand the concept of ‘piezoelectricity’, which is, in essence, the coupling of a material’s mechanical and electrical behaviour. When a piezoelectric material is squeezed, twisted or bent, electric charges can collect on its surfaces. Conversely, when it is subjected to a voltage drop it will mechanically deform.
What is nanopiezotronics?
How well do you really know your competitors?
Access the most comprehensive Company Profiles on the market, powered by GlobalData. Save hours of research. Gain competitive edge.
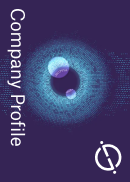
Thank you!
Your download email will arrive shortly
Not ready to buy yet? Download a free sample
We are confident about the unique quality of our Company Profiles. However, we want you to make the most beneficial decision for your business, so we offer a free sample that you can download by submitting the below form
By GlobalDataNanopiezotronics is the coupling of the piezoelectric and semi-conducting properties of miniscule nanowires and nanobelts, which can lead to the design and fabrication of miniature electronic devices, such as nanotransistors and nanodiodes and, more importantly, from the medical perspective, devices that can be implanted into the body to harness a person’s natural biomechanical or biochemical energy.
For Wang, who has been working on developing this nanogenerator technology since 2005, the possibilities are almost endless. The global market for manufactured nanotechnology goods (not just medical ones) has been estimated by analysts to have the potential to be worth some $1.6 trillion by 2013.
Within the medical devices, biomechanics and biochemical arena, it could be using the energy generated by a heartbeat, blood flow or a patient’s breathing, but it could just as easily also mean harvesting the low-frequency emissions that are obtained from irregular mechanical motion, such as muscle stretching or the vibration of vocal cords.
What’s more, when you take into account that battery-powered medical devices, such as pacemakers, inevitably have a finite life and, therefore, need replacing around every 15 years, a process that requires yet more invasive surgery, the benefits of going battery-less become clear.
On top of this, nanogenerators do not use toxic-heavy metals in their manufacture (although piezoelectric materials do), therefore potentially making them more environmentally friendly to use, as well as safer for implanting into the body.
When it comes to manufacturing processes, they can be made at temperatures lower than the boiling point of water, and even below those required for producing standard electronics.
This, potentially, saves on the cost of manufacture and, in turn, makes them more attractive for large-scale, mainstream production.
In vivo nanogenerators
Among its innovations, the Georgia Tech team has demonstrated how it is possible to convert biomechanical energy into electricity through the use of an in-vitro muscle-movement-driven nanogenerator. Of course, going in vivo is a whole different ball-game, involving the need to address issues such as toxicity and biocompatibility, as Wang concedes.
“The area of medical devices is complex. Because you need to get FDA approval, you have to prove a device’s reliability and safety, and there are many very sophisticated industries that you have to work with,” he explains. “Current technology is very heavily reliant on battery power, either rechargeable or replaceable. So if we can instead harvest the energy from biomechanical and biochemical sources, that will be a very friendly energy source and, potentially, serve a lot of needs.
“There is a lot of biomechanical and biochemical energy inside our bodies. It is possible to convert, for example, glucose into biofuel cells and harvest that chemical energy. These days devices are becoming smaller and smaller and have lower power consumption, so this type of innovation meets the needs of the technology; it fits perfectly with medical power needs.”
When it comes to in-vivo devices, the Georgia Tech team has already successfully implanted a nanogenerator into a live rat to generate electricity from its heart. In this experiment, zinc-oxide nanowires were deposited onto a flexible polymer substrate, so allowing the nanowires to bend in a variety of ways.
This device was then sealed in a polymer shell to shield it from body fluids, as well as to ensure that any electricity measured was generated by the device and not background interference. This 2mm x 5mm rectangular device, barely visible to the naked eye, was then attached to the rat’s diaphragm muscle using tissue adhesive.
With each breath, the implant stretched and twisted, thereby deforming the nanowires and, in the process, generating up to four picoamps of current at a potential of two millivolts (mV).
This experiment was followed by the implantation of a similar device on a different rat’s heart, an experiment that generated 30 picoamps at 3mV. While this sort of energy generation may not sound significant, Wang argues that, after some scaling-up activity has been achieved, it should, in principle, be possible to generate enough power for simple implantable nanosensors. This technology becomes particularly feasible when the device or sensor requires only a modest amount of power to function and/or does not need a continuous supply of power to operate.
“If you are looking to monitor blood pressure or glucose levels, it could be ideal as a sensor,” Wang says.
Potential of nanopiezotronics
Georgia Tech is not the only centre pioneering this type of technology. In 2009, researchers at NASA’s Glenn Research Center in Cleveland, Ohio, implanted a ceramic piezoelectric generator into a rabbit’s quadriceps muscle, in the process successfully generating enough electricity to power a simulator attached to the same muscle.
When scaled up to incorporate larger muscles and circuitry improvements, this technique, the researchers argued at the time, would also lead to increased available power.
“An implanted, self-replenishing power source has the potential to augment implanted battery or transcutaneously powered electronic medical devices,” the researchers concluded.
For Wang, the recent advances achieved by his team have made him much more optimistic that, while still very much a cutting-edge, lab-based technology at the moment, nanopiezotronics has the potential in the not-too-distant future to become a reality within the clinical and healthcare environment.
Wang cites the fact that New Scientist magazine recently rated nanopiezotronics as one of its top ten innovations to watch out for in the next ten to 30 years.
“It has said nanopiezotronics will be as important as the invention of the mobile phone,” he said.
The research by the institute also shows that it is now perfectly feasible to ‘scavenge’ biomechanical and biochemical energy from within a body, something that represents an important step towards implantable, self-powered nanosystems, he argues.
“I can see it starting to be applied within three to five years,” he says. “I used to say maybe six years, but the progress we have made this year has been gigantic, in particular around using biomechanical energy to light up LED lights, for example. It could be used for a lot of medical devices.
“This is the sort of platform technology that will be able to meet the energy needs of a lot of smaller medical devices. I think you will start to see its application in areas such as diabetes control and monitoring of blood pressure.
“So it may be a case of implanting a medical device that enables you to detect your blood sugar levels and then transmit that information to a watch-like device which can catch that data and display it, with the original medical device being powered by biomechanical energy,” he says.