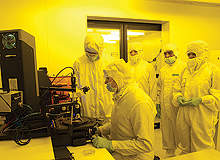
Microfluidics has the potential to develop into one of medical devices’ most exciting arenas. Its application ranges from mobile assays for disaster zones to bed-side diagnostic tools that deliver results faster than a lab. But outstanding innovation of the academic sectors often fails to make a connection with the industry.
In May 2010 the University of Cincinnati was given a $3m grant to set up the Center for Microfluidic Innovation (OCMI) and Ian Papautsky, a pioneer in the field, took the helm. The subject has been neglected by the scientific press for years, but is about to enjoy a big revival of interest thanks to investment in a new discovery. Papautsky and colleagues at the University of Cincinnati published a paper in the March edition of the journal, Lab on a Chip, about virtual electro-wetting channels.
The team has found a way to transport liquid electronically in virtual channels. Channels are formed by the application of voltage to polymer posts in a device. The posts, coated with a conductor and a hydrophobic dielectric, can perform electro-wetting, which means that a voltage can change the surface tension of a liquid, making it almost hydrophilic.
The channels are unique in that they can hold their channel geometry even in the absence of voltage. Arrayed controls combine the advantages of programmable electro-wetting with the continous channel functionality on a single device.
Virtual channel systems
See Also:
For the past three decades the focus in microfluidics has been on developing the science and technology of lab-on-a-chip assays. At first the devices were typically made from glass, which required specialised equipment to etch channels, so eventually costs drove the industry towards using plastic. Conventional microfluidic devices were made of two substrates stuck together, one flat and one with channels through which liquids could flow.
How well do you really know your competitors?
Access the most comprehensive Company Profiles on the market, powered by GlobalData. Save hours of research. Gain competitive edge.
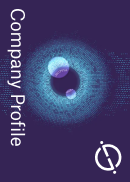
Thank you!
Your download email will arrive shortly
Not ready to buy yet? Download a free sample
We are confident about the unique quality of our Company Profiles. However, we want you to make the most beneficial decision for your business, so we offer a free sample that you can download by submitting the below form
By GlobalDataBut these structures conformed to and were limited by their designs. A device to detect one particular biomarker would have a certain design, and a different device would be required to look for a different biomarker.
Virtual channel systems bring conventional and digital microfluidics together by combining the rigidity of conventional systems with the mutability of digital. Papautsky describes the virtual channels interface like an Etch A Sketch.
“The basic idea is that you use electric fields to draw out liquid, not just in drops, but in a stream,” he explains. “This creates continuously flowing virtual channels. You can then redraw microfluidic networks like an etch-a-sketch – draw a network, erase it and draw a new one.”
The devices will be fronted with the same displays used for mobile telephones, which could be interfaced with the microfluidic layer. Costs will initially be much higher, but ultimately the technology could be disposable. Components such as the displays are already relatively cheap and LOAC devices could soon cost less than $10 a piece.
“We’re not there yet,” says Papautsky. “Right now we’re able to draw straight channels out of a droplet, and we’re still working on the electric interface. If we’re able to interface the posts, we can make the liquid go where we want it to.”
The field has already developed the right expertise and so the focus can now shift to device creation.
“Think about glucose testing as an example. Years ago analysis was a lot more complicated and samples needed to go to a lab. Today, you could go to a drug store and prick your finger and you’d have your analysis.”
The glucose assay combines the three established principles of commercial devices: cheap, mobile and disposable.
OCMI goals
OCMI’s priority is making assays work faster. Papautsky’s experience from working with clinicians is that they are not primarily interested in bedside technology nor are they clamouring for mobile. Most doctors prioritise speed over all other aspects.
Papautsky and his colleague Jason Heikenfeld are working on a project that will go to market in 2011. Siloam commissioned a next-generation micro-plate for running enzyme-linked immuno-absorbent assays (ELISA). This product uses microfluidics to substantially reduce sample volume and increase surface to area ratio. Reactions can take place faster and get more fluorescent signal, plus the cost of running the assay is a tenth of previous models.
The OCMI is also working on a project for the National Institute of Health to develop a lab-on-a-chip for rapid analysis of zinc levels in blood. The metal is vital to the healthy working of the human metabolism and a lack of it is a symptom of septic shock.
Analysing blood only takes a few hours, but collecting a sample, sending it off to the lab and getting the results back could take 48 hours or more.
“For a patient in the ICU that’s too long,” Papautsky says. “Our goal was to reduce analysis time. Instead of sending the sample off to a centralised lab, analysis could be done in the hospital. It might not be done in minutes – it could still take a couple of hours – but by cutting out the shipping and sample preparation time it’s a much more manageable time scale for a patient.”
Pros and cons of lab-on-a-chip
There are key advantages to using lab-on-a-chip technology. Firstly, small channels need only small volumes of chemicals, so the cost of components is low. Secondly, small channels also mean a high surface to volume ratio. Chemical reactions speed up and there’s a greater current with which to detect electric chemicals.
A third advantage to the small channels is that designers could potentially create a highly parallel system. Instead of one system, you could run up to a hundred on the same device. It would take a long time to set up a large stock but it could be managed easily with the use of a robotic pipette.
Most clinicians are happy with desktop assays, as Papautsky points out, but there has been a lot of hype about building smaller and smaller devices. He thinks that the obsession with size has gone far enough.
“You need to have practical limits,” he says. “You can go too small. In micro-channels we have millimetre-wide channels, which are relatively wide – 1mm is a volume of 1 microlitre and it contains about a billion molecules. But 1 micrometre cubed is a femtolitre and it contains just a few molecules.”
Not only are reactions on that scale problematic, but assays on this scale are more expensive. In the field, mobile applications would be more useful than micro-devices, so size is still a moot factor. A small device, such as an iPhone or a glucose meter, could be plugged in to the mains and perform basic analysis wherever it went.
While it might not have a huge capability, something like a water quality assay could be a huge health precaution for the community it served.
Fast-working devices would be particularly relevant to walk-in clinics, for example in refugee camps, because patients require on-the-spot diagnosis. Assays could also be plugged into broadband and link to a central database.
They are designed to work autonomously. Papautsky says that the primary challenge that academics in this area face is the lack of technical standards.
“This industry started out in the world of semi-conductors where they have standard methods of processing silicon and depositing layers regardless of whether they are making iPhone processors, cell phone processors or whatever,” he explains.
At present labs making microfluidic devices have a wide range of technology at their disposal, but still struggle to push their developments through to industrial partners. Papautsky encourages those in the market to approach academic partners.
“Now is the time to step up,” he says. “We know how to fabricate, so we can take over and start developing specialised assays and actual products.”
More often than not, labs simply work with what equipment they have to hand. For example, while one lab with a hot embossing system might manufacture a device in one fashion, a lab without the embossing system would make it totally differently. Lack of standards has become an acknowledged problem and labs are crying out for technological unity.
“There are efforts to standardise and it will start to happen more and more as we transition into the industry,” says Papautsky.
In fact he thinks that the future success of the industry depends on it. Unified design and manufacture would normalise costs, which would not only stabilise the industry, but also make it easier and quicker to introduce new devices to the market.
“If you have a lab-on-a-chip to detect biomarker A, you could rapidly adapt the same device to detect biomarker B without setting up entirely new manufacturing facilities,” he says.
Microfluidic potential
The goal at the OCMI, according to Papautsky, is to facilitate the transition of microfluidic and LOAC devices and technologies to industry. One practical example of how it will do this is by promoting the use of six-inch wafers in assay production.
In most academic labs, three or four-inch wafers are the norm for processing relatively small sample sizes. Just as it is in the semi-conductor industry, using larger wafer sizes in the microfluidic industry cuts costs because the batch process is higher. This particular transition would benefit businesses that want a production line or a small line of prototypes.
Microfluidics has yet to reach its potential in terms of application but has come a long way since the initial bubble of interest. Virtual channel technology promises to draw the scientific community back after a ten-year break.
“I think we’re at a point now that the technologies are mature enough to transition from academia to industry,” says Papautsky.
With so much potential for assays, for the technology and its application, there’s no better time to forge partnerships in this field.